
Engineering better stem cell therapies to turn back the clock on aging muscles
By Geoffrey Giller
When most of us think of stem cells, we think of cells that are present early in our development and specialize to become bone, brain or a myriad of other tissues in our bodies. But in each of us, a small but significant fraction of our cells are, in fact, still stem cells.
One type of these stem cells sits quietly near our muscle fibers—quietly, that is, until those muscles are damaged. That damage may come from strenuous exercise, like weight-lifting; or, it can be the consequence of a traumatic injury. Either way, in response to an alerting signal, these muscle stem cells, sometimes called satellite cells, wake up and get to work.
The way those cells decide what to do when they’re awake, though, is a complex process. But Ben Cosgrove, an assistant professor at the Meinig School of Biomedical Engineering at Cornell, aims to disentangle and map out that process, figuring out what makes those muscle stem cells tick—and harnessing that information to fight diseases and combat the effects of aging.
“As we age... the ability of these cells to respond to muscle injuries declines,” Cosgrove explains. That decline is precipitous for people over about age 70. “Fundamentally, we’re interested in harnessing the ability of the stem cell, through engineering approaches, to enhance its contribution to muscle repair, and hopefully to slow the progression of aging and other degenerative diseases,” he says.
Stem cells, by definition, can specialize into a vast array of different cell types that perform specific functions. Muscle stem cells, as they divide and multiply after an injury, can either become new, more specialized muscle cells, or they can maintain their identity as stem cells in a process called self-renewal. One of the reasons muscle repair declines as we get older, Cosgrove says, is that later in life, ever greater fractions of these muscle stem cells become dysfunctional and lose control of the self-renewal process.
In younger, more healthy tissue, muscle stem cells create more of themselves in order to maintain a pool of cells that can respond to future injuries. He likens it to the source of a river, which needs to be replenished so that the river can continue to flow. In older people—or in older mice, which Cosgrove’s lab uses as its study organism—self-renewal happens less and less, says Cosgrove. Eventually, the source of those cells can start to dry up, leaving muscles unable to effectively respond to injuries.
That’s where Cosgrove’s work comes in. “We’re often interested, as engineers, in being able to control these cell-fate transitions,” he says. Is it possible to engineer the muscle stem cells of older people so that the cells replenish themselves more effectively? “If we could better understand what regulates their fate decisions, we could engineer therapies that target and control them,” Cosgrove says.
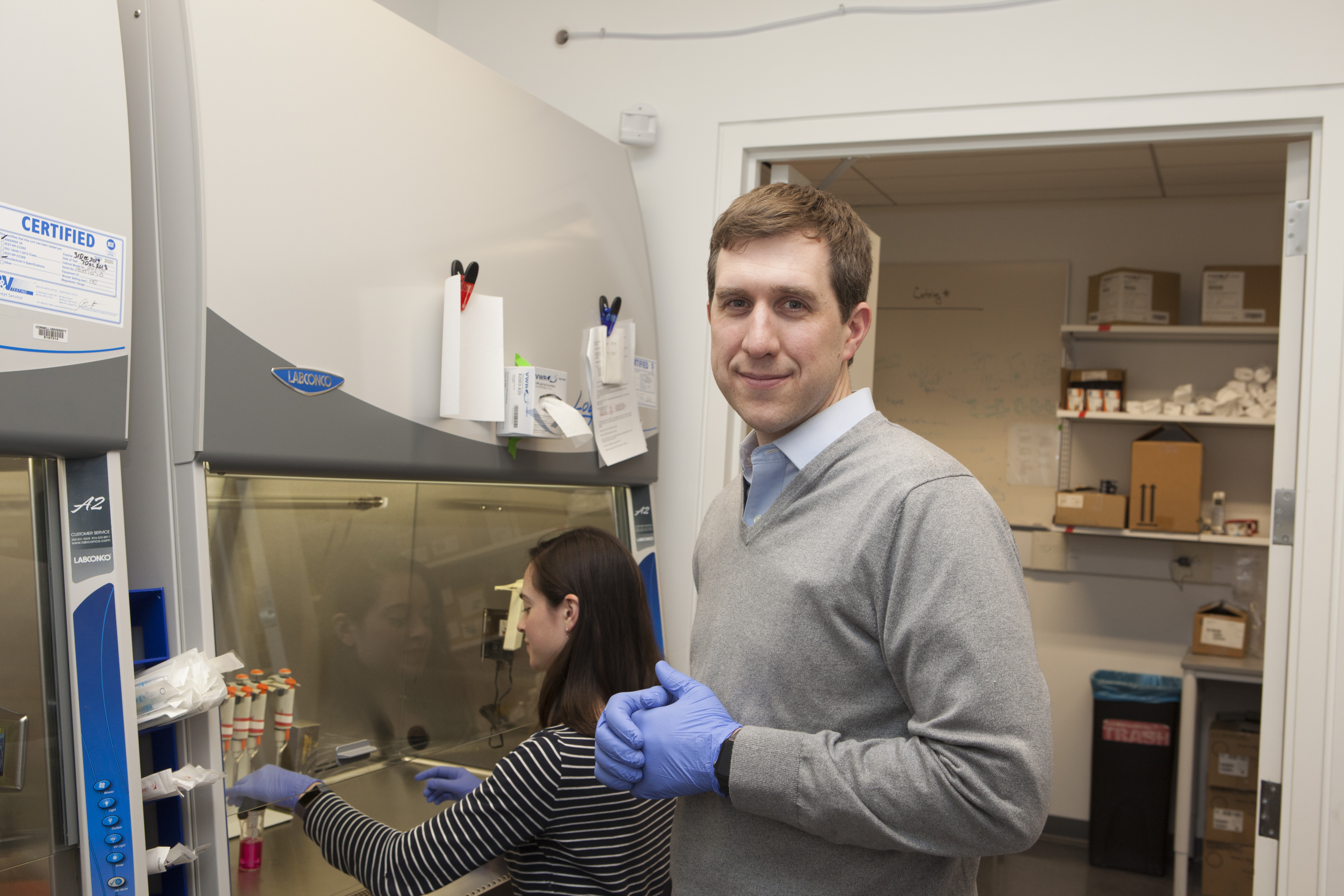
Along with collaborator Iwijn De Vlaminck, assistant professor of biomedical engineering, one approach Cosgrove has taken in figuring out which factors lead to self-renewal has been to work backwards, taking injured muscle tissue and seeing how muscle stem cells change in response to the injury. That process involves taking muscle from a mouse and digesting it down into individual cells. Then, each cell’s transcriptome—that is, measuring the level of every active gene in that cell as reflected by messenger RNA it generates—is analyzed and used to creating a map of the dozen or so cell types in muscle tissue.
“From this cell atlas,” Cosgrove says, he and his colleagues can “infer what the roadmap is—how the cells are talking with each other.” Of course, this is no easy feat and is all happening within “a cacophony of different communication factors,” he says.
Andrea De Micheli, a fourth-year Ph.D. student in Cosgrove’s lab, has recently been building this cell atlas. He takes tissue samples from injured mice and analyzes the transcriptome from thousands of individual cells, looking to see which genes are activated or deactivated within each type of cell. In particular, he wants to figure out if there are what he calls “sub-states” of cells—that is, states that have not yet been identified that might exist in between previously-identified ones. Those newly-identified states, he says, will help generate new hypotheses as to how the cells are interacting and what signals the stem cells might be responding to, which can then be tested in the lab.
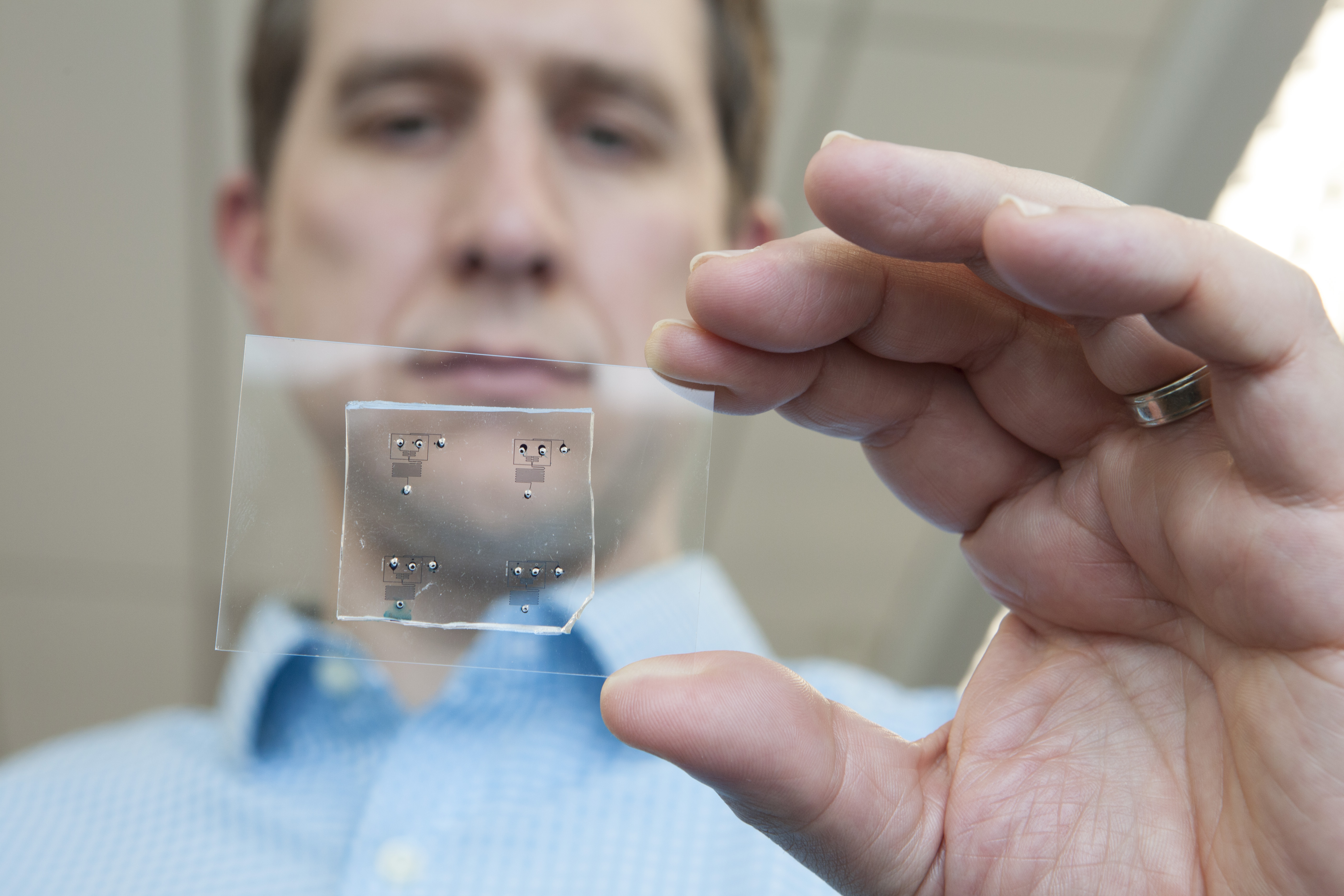
Another major undertaking in the Cosgrove Lab is the creation of new manufacturing techniques and materials that will eventually facilitate the translation of this work into human therapies.
One challenge to using muscle stem cells therapeutically, Cosgrove says, is creating enough cells to actually have an effect when they’re injected. Right now, with existing technologies on the market, “You’re orders of magnitude below where you need to be, in terms of the yield,” he says. Without enough stem cells, there’s no real effect. The way these therapies could work is that cells can be injected directly into the muscles of patients with, for example, muscular dystrophy—a degenerative disease that causes muscles to weaken and lose function, including ones essential for breathing.
“Could we generate enough cells that we could replenish, for example, the diaphragm muscle?” Cosgrove asks. (The mutated genes responsible for the disease would also have to be corrected for the therapy to work, which is another area that his lab is focused on.) Cosgrove also aims to treat the muscles that control swallowing—which can weaken in aging, having a major impact on quality of life—with engineered muscle stem cell therapies.
Cosgrove and his students have come up with a new technique that, he says, produces significantly more muscle stem cells than the methods commercially available. They use a cocktail of factors that can get muscle stem cells to both multiply and self-renew—a cocktail whose ingredients he derived in part from the cell atlas work.
On a separate but intersecting track, Cosgrove is also developing a new material that can serve as a scaffold on which the multiplying muscle stem cells can thrive. Cosgrove has created tiny, biodegradable beads that can serve as a protective capsule for the cells and can be injected directly into diseased muscle tissues. Studies in mice have shown that stem cells injected this way can migrate within a given muscle and replenish its stem cells. Ultimately, the goal, Cosgrove says, is for these two approaches to complement each other: the cocktail of factors creates enough muscle stem cells to make a difference when injected into a patient, and the beads serve as a specialized delivery system for those cells.
Emily Laurilliard, a first-year Ph.D. student in the lab, says she was drawn in part to Cosgrove’s work because she had worked with biomaterials as an undergraduate. She’s intrigued by the prospect of bridging biomaterials, engineering and stem cells.
“Our body has these little pockets of these adult stem cells that have this great potential, which is really exciting,” Laurilliard says. “It’s kind of cool that our bodies have this built-in way to heal itself.”
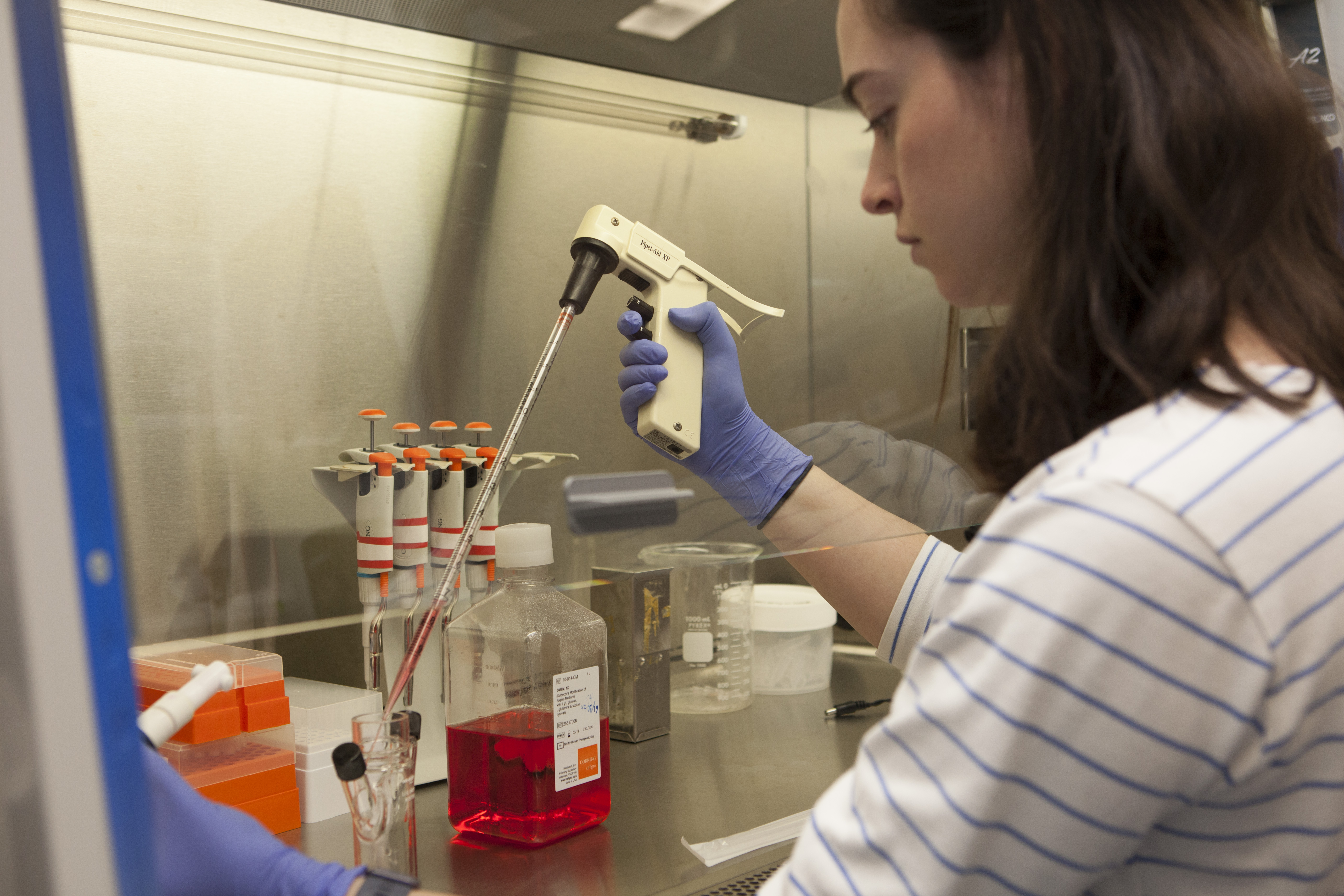
While the work in his lab is promising, Cosgrove cautions that the use of this research in humans is still a long way off—likely a decade or more, after the engineering is worked out and the eventual treatments go through the regulatory process. But eventually, “if we can understand how the cells are thinking in their complicated environments,” Cosgrove says, “we can engineer better therapies.”